Brian B. Laird
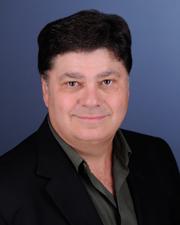
- Professor
- Courtesy Professor - Chemical & Petroleum Engineering
- Courtesy Professor - Physics & Astronomy
Contact Info
Lawrence
1567 Irving Hill Rd
Lawrence, KS 66045
Personal Links
Education —
Specialization
Computational Materials Science and Applied Statistical Mechanics
Research —
Theoretical and computational chemistry
The ultimate goal of materials chemistry is the understanding of the macroscopic properties of materials in terms of the microscopic molecular interactions. This is a common theme in all of the natural sciences. The differences between the various scientific disciplines (chemistry, biology, physics, materials sciences, etc.) often disappear as the traditional macroscopic phenomenology is replaced by a more molecular approach. At present, most of what is known about the chemical and physical properties of materials is still largely empirical, especially in the case of amorphous, macromolecular or interfacial systems. The development of a microscopic theoretical description for a variety of such complex systems is the primary focus of our research. Several representative projects are listed below. They are all projects in which great advantage will be gained by exploiting the natural symbiosis between analytical and computer-simulation techniques.
1) Crystal-melt interfaces of complex systems:
The structure and dynamics of an interface between a crystal and its melt are of paramount importance in studies of crystal growth and nucleation. Experimental study is difficult as such an interface lies sandwiched between two dense phases, and experimental data is lacking, increasing the value of computer simulations to the study of such systems. Most previous studies have involved simple, one-component model systems. Using molecular-dynamics computer simulations and classical density-functional theories, we are currently concentrating on more complex systems such as multicomponent systems (for example, alloys) and molecular systems, such as succinonitrile and pivalic acid, for which extensive data relating to crystal growth and interfacial properties have been collected from microgravity experiments aboard the Space Shuttle.
2) Fundamental Investigations into Gas Expanded Solvent Media for Green Chemistry:
This project is part of the research mission of the newly formed Center for Environmentally Beneficial Catalysis, an NSF funded Engineering Research Center headquartered at the University of Kansas. In this project we seek a fundamental understanding, through molecular simulation, of the thermodynamics, transport properties and molecular-level structure of gas-expanded solvent systems - a new class of solvent media in which a traditional industrial organic solvents, such as acetonitrile, are expanded by an order of magnitude in volume (without phase separation) by 2.
In these new media, dense CO2 significantly replaces the traditional solvent (up to 80% by volume), such that the retained solvent maintains the catalyst solubility and other advantages provided by the traditional solvent (for example, rate enhancement due to polarity). Further, solubility is increased for O2 Thus, gas-expanded solvents combine the environmentally beneficial nature of supercritical (sc) solvents (such as sc-CO2) and the advantages of traditional solvents in an optimal manner, thereby deriving many reaction and environmental/economic advantages not possible with either the neat solvent or sc-fluid. Due to their novelty, these gas-expanded solvent systems have received little attention from the molecular simulation community. To complement the current experimental effort, this project represents a parallel program of modeling efforts to
- gain a fundamental molecular-level understanding of the physico-chemical properties of gas-expanded solvents, with particular attention to those systems utilizing CO2 as the expansion gas. provide guidance to the experimental program to rationally chose optimum solvents
- provide guidance to the experimental program in the optimization of catalyst performance in expanded media.
3) Algorithms for Molecular Modeling:
Molecular-dynamics computer simulation has become an invaluable tool in chemistry, chemical engineering, physics, materials science and biology; however, its uses are still limited by the relatively small system sizes and short time scales that can be simulated at present. Progress in this area therefore comes from advances in computer technology and in the development of efficient and stable algorithms. The latter is the goal of an ongoing multidisciplinary project in collaboration with Prof. Ben Leimkuhler, an applied mathematician at the University of Leicester.
Selected Publications —
Davidchack, R.L., Elmajdoub, A.A., and Laird, B.B. Analysis of probability of inserting a hard spherical particle with small diameter in hard-sphere fluid. J. Chem. Phys. 159, 184503 (2023). https://doi.org/10.1063/5.0170928
Nguyen, N.P., and Laird, B.B. Generation of Amorphous Silica Surfaces with Controlled Roughness. J. Phys. Chem. A, 2023, 127, 9831-9841. https://doi.org/10.1021/acs.jpca.3c04955
Abascal, J.L.F., Laird, B.B., Noya, E.G., and Stebe, K.J. Fluids meet solids. J. Chem. Phys. 158, 220401 (2023). https://doi.org/10.1063/5.0156357
Zhang, X., Laird, B.B., Liang, H., Lu, W., Yu, Z., Ma, X., Cheng, Y., and Yang, Y., Atomistic characterization of the SiO2 high-density liquid/low-density liquid interface. J. Chem. Phys. 157, 134703 (2022) https://doi.org/10.1063/5.0107674
Seth C. Martin, Hendrik Hansen-Goos, Roland Roth, and Brian B. Laird, "Inside and out: Surface thermodynamics from positive to negative curvature", J. Chem. Phys. 157, 054702 (2022) https://doi.org/10.1063/5.0099295
Ruslan L. Davidchack and Brian B. Laird , "Chemical potential and surface free energy of a hard spherical particle in hard-sphere fluid over the full range of particle diameters", J. Chem. Phys. 157, 074701 (2022) https://doi.org/10.1063/5.0100073
Xin Zhang, Wenliang Lu, Zun Liang, Yashen Wang, Songtai Lv, Hongtao Liang, Brian B. Laird, and Yang Yang , "Local collective dynamics at equilibrium BCC crystal–melt interfaces", J. Chem. Phys. 157, 084709 (2022) https://doi.org/10.1063/5.0101348
Wimalasiri, P.N., et al., Amorphous Silica Slab Models with Variable Surface Roughness and Silanol Density for Use in Simulations of Dynamics and Catalysis. Journal of Physical Chemistry C, 2021. 125(42): p. 23418-23434.
Piskulich, Z.A. and B.B. Laird, Molecular Simulations of Phase Equilibria and Transport Properties in a Model CO2-Expanded Lithium Perchlorate Electrolyte. Journal of Physical Chemistry B, 2021. 125(32): p. 9341-9349.
Xu, X.Q., et al., Kinetics of Crystallization and Orientational Ordering in Dipolar Particle Systems. Crystal Growth & Design, 2020. 20(12): p. 7862-7873.
Martin, S.C., H. Hansen-Goos, and B.B. Laird, Surface Free Energy of a Hard-Disk Fluid at Curved Hard Walls: Theory and Simulation. Journal of Physical Chemistry B, 2020. 124(36): p. 7938-7947.
Kern, J.L., P.R. Barry, and B.B. Laird, Characterization of the Al-Ga solid-liquid interface using classical and ab initio molecular dynamics simulation. Physical Review Materials, 2020. 4(4): p. 9.
Palafox-Hernandez, J.P., et al., Pressure and Temperature Tuning of Gas-Expanded Liquid Structure and Dynamics. Journal of Physical Chemistry B, 2019. 123(13): p. 2915-2924.
Davidchack, R.L. and B.B. Laird, Surface free energy of a hard-sphere fluid at curved walls: Deviations from morphometric thermodynamics (vol 149, 174706, 2018). Journal of Chemical Physics, 2019. 150(6): p. 1.
Paganini, I.E., et al., Properties of the hard-sphere fluid at a planar wall using virial series and molecular-dynamics simulation. Journal of Chemical Physics, 2018. 149(1): p. 8.
Martin, S.C., et al., Thermodynamics of the hard-disk fluid at a planar hard wall: Generalized scaled-particle theory and Monte Carlo simulation. Journal of Chemical Physics, 2018. 149(8): p. 8.
Davidchack, R.L. and B.B. Laird, Surface free energy of a hard-sphere fluid at curved walls: Deviations from morphometric thermodynamics. Journal of Chemical Physics, 2018. 149(17): p. 8.